Africa’s energy access issues could be solved with solar-plus-storage systems: With over 600 million Africans lacking access to reliable electricity, solar paired with storage offers a transformative solution, especially in rural and off-grid regions.
Falling costs in solar and storage technology are making renewable energy more competitive: The levelized cost of energy (LCOE) for solar-plus-storage systems is projected to drop to $0.12 per kWh by 2030, making it competitive with fossil fuel-based energy sources.
Concentrate Solar Power (CSP) systems provide longer-duration storage but face higher capital costs: While CSP systems, like Morocco’s Noor Ouarzazate plant, can store energy for up to 7 hours, they require higher initial investments and more water than PV-based solar plants.
In Africa, approximately 600 million people still lack access to electricity, with the majority concentrated in sub-Saharan Africa. This represents about 43% of the continent’s population, with rural areas being the most affected. For instance, in countries like Nigeria and the Democratic Republic of Congo, energy poverty remains pervasive, with access rates as low as 20% in some regions. [1]
Fig. 1 Accessibility of electricity in Africa
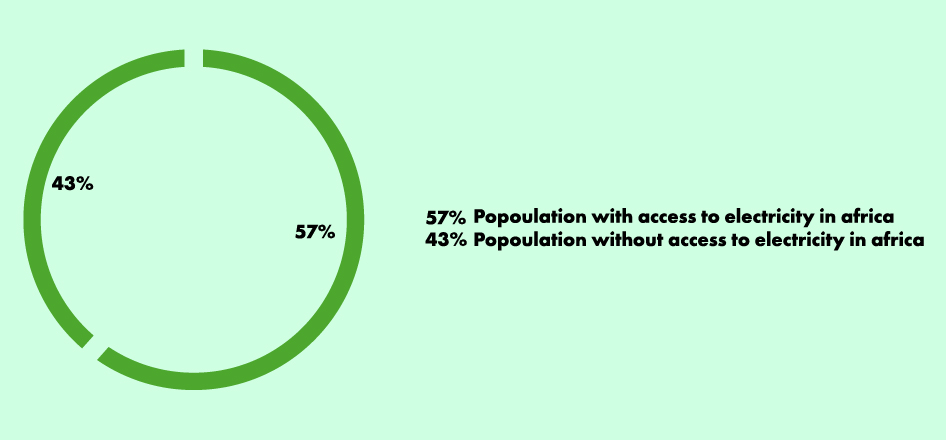
Africa is uniquely positioned to address this challenge through solar power, as the continent is home to 60% of the world’s best solar resources. Despite this vast potential, the installed solar energy capacity in Africa only accounts for 1-1.4% of the global installed solar capacity. To bridge the electrification gap, decentralized solar grids, particularly those paired with battery storage, offer a scalable solution. These systems can bring reliable, renewable energy to remote areas where extending traditional grids is impractical. [1,2]
Fig. 2: According to the International Energy Agency (IEA), Africa has 60% of the world’s best solar resources, but only 1% of solar generation capacity.
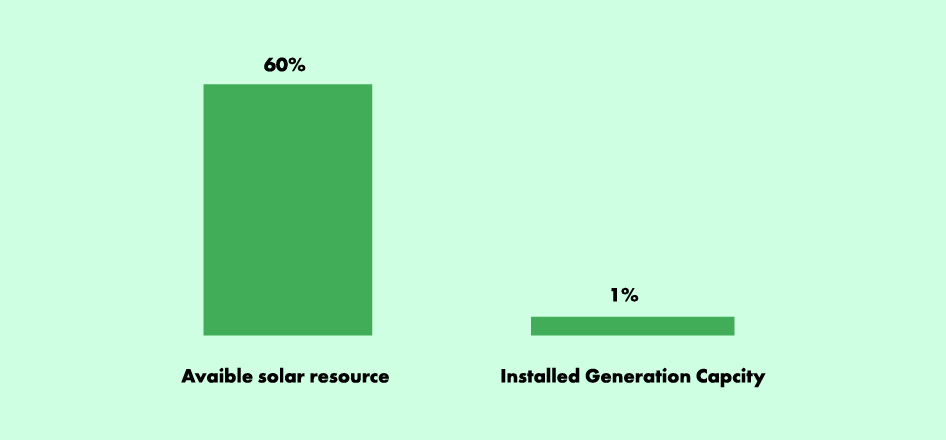
Load Following and Energy Dispatch with Solar-plus-storage
The intermittency of solar energy has historically been a challenge, as it relies on sunlight to generate power, creating peaks during the day but none at night. Battery storage solves this by storing excess solar energy produced during the day for use during the evening and night. This addresses the need for “dispatchable power”—reliable electricity that can be supplied on demand. ‘Dispatchable’ means that it can be switched on or off, or ramped up or down, depending upon demand. For instance, the TotalEnergies and Hydra Storage Holding plant under construction in South Africa, is set to generate 216 MW of solar energy and store 500 MWh in batteries with a discharge speed of 75 MW. It will be providing a constant electric supply at 75 MW from 5:00 AM to 9:30 PM throughout the year [10]. Such projects demonstrate how solar + storage systems can provide energy even during peak demand hours, making them vital for closing Africa’s energy gap.
In other words, in order for a renewable energy solution like solar to be able to match conventional generation capacity, they need to be able to follow the load demand curve during the day, especially during peak hours. [5] Load following is the ability of a plant to match the load pattern over the course of an operating day. The key requirement is being able to provide the power when and as needed by the system load. In this sense, the power plant ‘follows’ the load. For solar power plants, this is challenging due to their reliance on sunlight, which peaks in the middle of the day and drops to zero at night. Without storage, solar plants can only generate electricity during these high-sunlight hours, leaving a gap between solar energy generation and peak demand in the evening (commonly referred to as the “duck curve”).The duck curve represents the mismatch between electricity demand and solar generation: in the middle of the day, there’s overgeneration from solar power, and in the evening, when demand spikes, solar power production plummets. This results in the need for expensive, fast-ramping energy sources like gas to meet the demand, especially during the evening “neck” of the curve when solar output is zero but demand is high.
In West African countries, the demand profile is slightly different, and the evening peak is a more significant issue than midday overgeneration. As seen in countries like Ghana and Nigeria, peak demand tends to occur during the evening, driven by lighting and cooling needs in residential areas, while industrial demand remains more consistent throughout the day. For example, Ghana’s 2012 load profile shows significant peaks in the evening, a pattern that is expected to persist as electricity access improves in the region. [21, 22] While a “duck curve” scenario is not common in West Africa due to lower midday demand and less penetration of solar energy compared to regions like California, the challenge remains to balance evening peaks with available generation. Solar-plus-storage systems are increasingly being deployed to ensure that energy generated during the day can be stored and used in the evening when demand is highest.
Fig. 4 System Load curve with and without storage where solar energy is involved
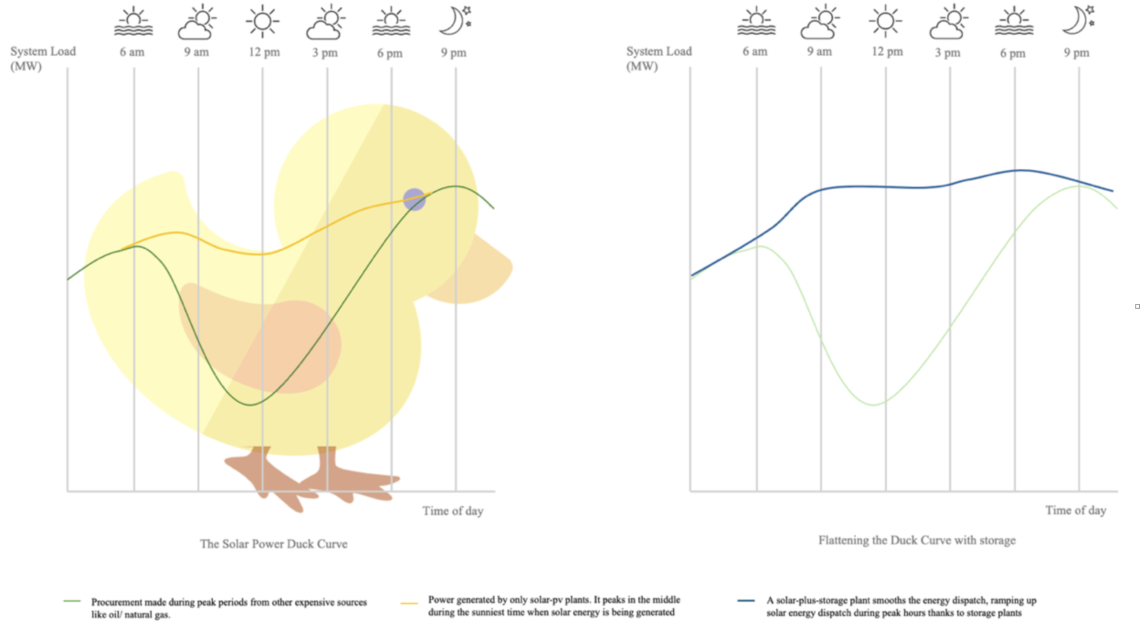
Flattening the Duck Curve with Battery Storage
Battery storage systems solve this issue by capturing excess solar energy generated during midday and dispatching it during peak demand in the evening. This flattens the duck curve by “filling in” the energy gap. In California, where the duck curve is a significant grid challenge, large-scale battery storage has grown from 0.2GW in 2018 to nearly 4.9 GW as of April 2023, with more storage capacity planned.[6]
When solar-plus-storage systems are in place, solar energy is stored during periods of low demand (midday), and this stored energy is then released in the evening or when demand spikes, smoothing out fluctuations in energy availability. The batteries used in these systems have specifications based on the power they can deliver (measured in MW) and the duration for which they can supply power (measured in MWh). For example, a system might be rated at 20 MW / 200 MWh, meaning it can provide 20 MW of power for 10 hours or 40 MW for 5 hours.
The EDF report from 2015[20] indicated that even at 60% renewable penetration, backup capacity, peaking units, and conventional thermal generation were essential to meet grid stability and demand-response needs. However, the recent solar-plus-storage projects in Africa (ref Table 1) show that energy storage can address many intermittency challenges, reducing the reliance on traditional thermal plants while providing reliable, continuous power.
The Kenhardt project, awarded in 2021 and operational by 2023, delivers 150 MW of power to the grid year-round from 5:00 am to 9:30 pm under a 20-year Power Purchase Agreement (PPA) with Eskom. This includes continuous dispatchable power beyond daylight hours, proving the reliability of solar-plus-storage systems. Similarly, the Noor Ouarzazate III plant in Morocco demonstrated stable performance during 10 days of testing, exporting 13.2 GWh of energy to the grid, even after sunset! In Mozambique, Globaleq’s solar-plus-storage system ensures a steady supply of electricity to 22,000 families via a 25-year PPA, displacing 172,000 tonnes of CO2 over the project’s lifetime. Ivory Coast’s Boundali plant and its 10MWh energy storage system ensures reliability of the plant’s production capacity (64 GWh/year), which can supply up to 30,000 households each year, while saving 27,000 tonnes of CO2. These projects highlight the ability of battery storage to extend the operational hours of solar power plants.
On smaller scale, it is harder to stabilize the reliability of renewable energy systems. For example, the Fekola Gold Mine in Mali operates 24 hours a day, and while it continues to rely on heavy fuel oil generators, the solar array allows three out of six generators to shut down during the day, reducing fuel consumption.
The role of frequency stability in solar-plus-storage systems: addressing inertia deficiency
Inertia, supplied by the rotating mass of traditional generators, is essential for stabilizing grid frequency during fluctuations in supply and demand. However, solar and wind plants, connected via power electronics, lack this inertia, making grids more vulnerable to frequency instability. To address this, Fast Frequency Response (FFR) is crucial. FFR is a near-instantaneous grid service where storage systems or other resources rapidly inject power to stabilize frequency after a disturbance. Various storage systems, such as battery energy storage systems (BESS), flywheels, and supercapacitors, can provide FFR due to their ability to respond within milliseconds to imbalances. These systems are particularly effective in compensating for the loss of inertia in high-renewable grids. FFR-enabled storage stabilizes the grid by reacting more quickly than conventional inertia, ensuring the grid remains reliable and responsive even with increased renewable energy penetration. Additionally, technologies like pumped hydro storage, though slower, can offer support for longer-duration balancing services. [23, 24]
The percentage of a power system that can realistically be powered by renewable energy sources is largely influenced by frequency stability and the system’s inertia. For grids with high renewable penetration (such as wind and solar), which do not provide natural inertia, managing frequency stability becomes more challenging. In general, systems with up to 50-60% renewable energy integration are manageable with current technologies like FFR, battery storage, and synthetic inertia from advanced grid technologies. [23, 24]
However, as renewable energy penetration rises above this threshold—especially beyond 70%—the system’s lack of inertia and the variability of renewables can cause instability if not compensated by storage or backup generation. For example, Long Duration Energy Storage (LDES) systems, such as pumped hydro storage and batteries, are increasingly used to extend this limit by providing grid balancing and stability services. FFR and similar technologies enable grids to operate with up to 60-70% renewables in systems with proper storage and balancing strategies in place. [23, 24]
Countries aiming for even higher renewable penetration (above 80%) typically require a combination of advanced storage solutions and grid management technologies to ensure reliability without the need for significant fossil-fuel backup. Therefore, while renewable energy can theoretically supply up to 70% or more of the grid with adequate storage and frequency response systems, the challenge of maintaining stability grows exponentially as the share of renewables increases. [23, 24]
Decreasing Costs of Solar PV and Li-Ion Batteries Are Driving Solar Installed Capacity
Scaling up solar-plus-storage systems across Africa requires significant investment. According to the International Energy Agency (IEA), Africa needs $190 billion per year in energy sector investments between 2026 and 2030, with two-thirds allocated to clean energy projects. While the capital costs for solar panels, battery storage, and grid infrastructure can be prohibitive for many African nations, the decreasing costs of solar PV and lithium-ion batteries are making these systems more financially feasible.
Since 1975, the cost of solar PV has dropped by 99%, making solar power increasingly affordable even in regions like Africa, where energy infrastructure is still developing. In 2023 alone, Africa added 3.7 GW of installed solar capacity.[2] As shown in fig.5, the cost of solar PV panels decreased from $105/W in 1975 to $0.2/W in 2020. At the same time, lithium-ion battery prices have followed a similarly dramatic downward trend. From $6,745 per kWh in 1991, prices fell to $156 per kWh by 2020, a 97% reduction. This drop in battery costs, driven by advancements in manufacturing and battery chemistry, has been critical in making solar-plus-storage systems increasingly viable and scalable. [7,8]
However, despite this decline in costs, financial models like public-private partnerships (PPP) are still essential to overcoming the initial capital requirements for these projects. Storage systems add significant cost, especially for long-duration storage. For example, alternatives like CSP (Concentrated Solar Power) are used to avoid some of these costs. The Noor Ouarzazate CSP project in Morocco has a levelized cost of energy (LCOE) of $0.14-$0.15 per kWh,[17] comparable to traditional power sources, highlighting the importance of diverse technological approaches to reduce costs.
Fig. 5 Price curves of PV solar panels and Li-ion Batteries depicting the same drop, just after a few years, making solar-plus-storage plants feasible [7,8]
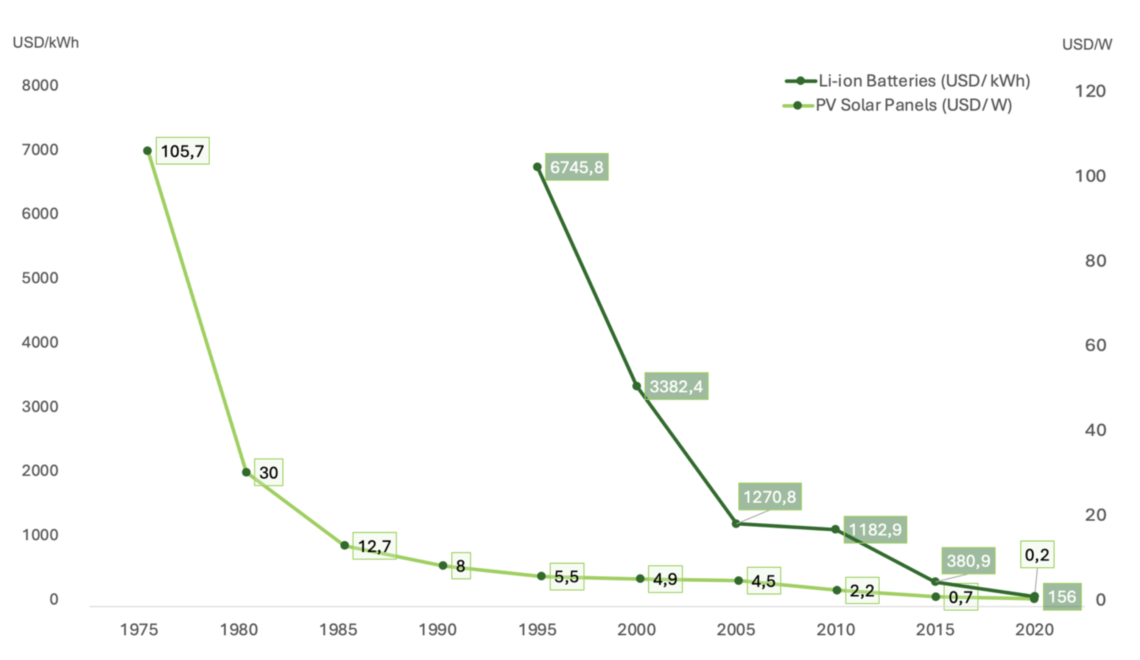
This massive reduction in costs has paved the way for large-scale solar PV deployment across the globe, 447GW of solar capacity was installed around the globe in 2023 alone. [9] While solar PV deployment is still in its early stages in many African countries, the rapid cost decline is enabling projects to accelerate, with an increasing number of initiatives focused on integrating solar-plus-storage systems. Table 1 gives a peak into some new solar-plus-storage projects in Africa.
Table 1: Africa’s Solar-plus-storage Projects
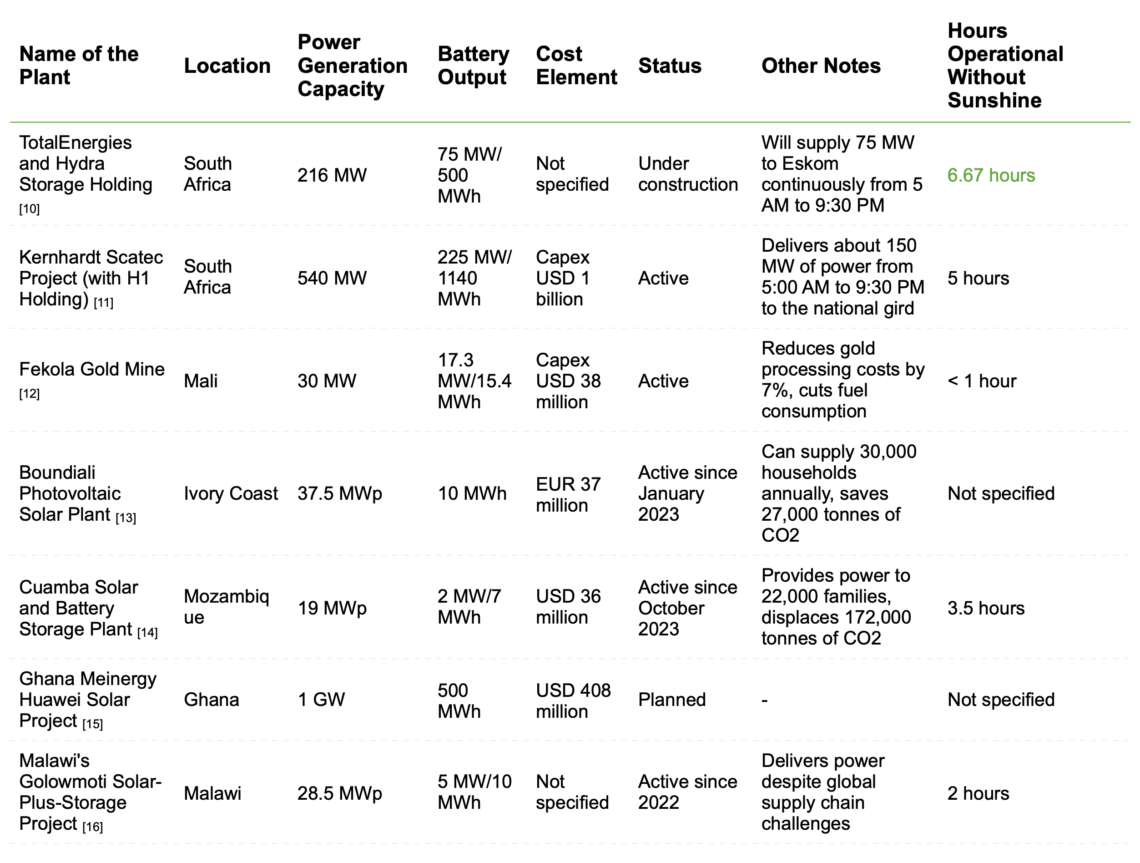
Looking ahead, battery cell prices are expected to fall even further, with industry leaders like CATL and BYD projecting a 50% reduction by 2024, bringing prices as low as $56/kWh. At that price, a 60 kWh battery that used to cost manufacturers $6,776.00 will cost just $3,388. These ongoing reductions are poised to make solar-plus-storage systems more accessible and competitive with traditional energy sources offering a clear path toward expanding Africa’s energy infrastructure. [7, 8]
Technology Advancements
Lithium-ion batteries have been the dominant storage technology, but thermal storage, as used in Concentrated Solar Power (CSP) plants, offers an alternative for long-duration storage at lower costs. CSP thermal storage systems, like that at the Noor Ouarzazate Power Plant can store energy for up to 7 hours. [17]
Table 2: Comparative Analysis for Solar + Storage vs CSP
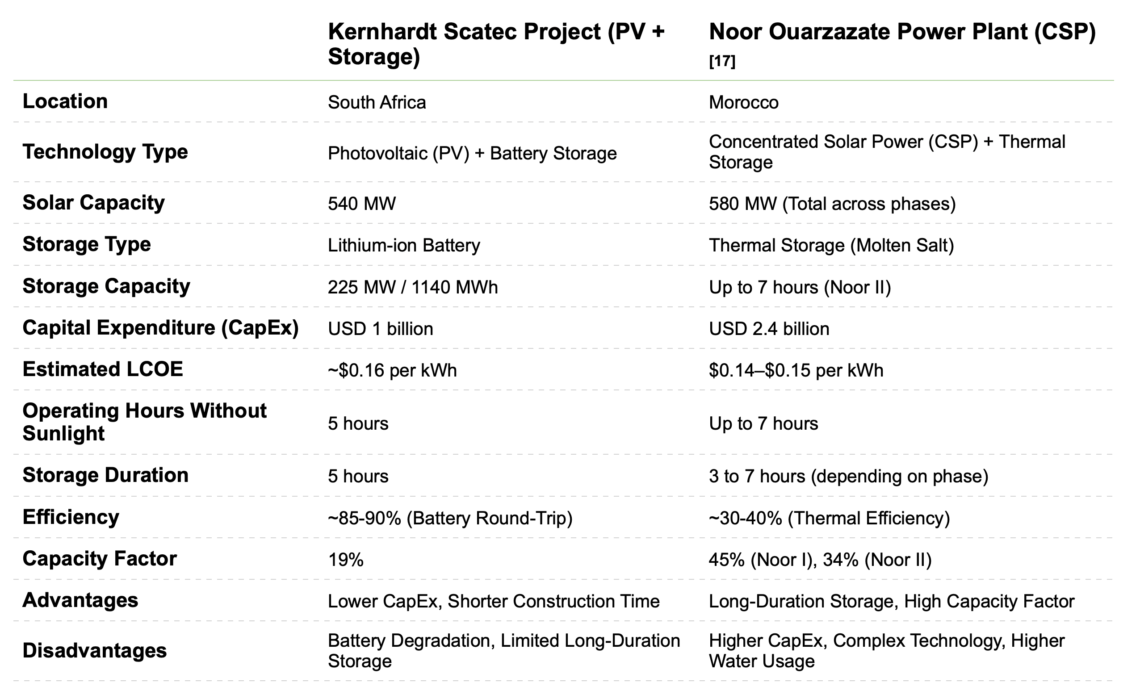
Battery storage, in itself, has evolved dramatically over the past decade, enabling solar systems to supply power even after sunset. The Arañuelo III solar-plus-storage system in Spain, commissioned in 2021, only had 3 MWh of battery capacity paired with 40 MW of solar generation [18]. This limited storage meant that its ability to supply energy after sunset was significantly constrained. More recently, in 2023, the Edwards & Sanborn project in California set a new standard for large-scale solar-plus-storage systems with an impressive 875 MW of solar PV and 3,287 MWh of battery storage. Spanning 4,600 acres, this project features 1.9 million PV modules and energy storage systems from top manufacturers like LG Chem, Samsung, and BYD, making it the largest solar-plus-storage project in the world.[19] In Africa, the Kernhardt Scatec Project also exemplifies this trend toward larger storage systems. The project stores 1140 MWh of energy, allowing it to deliver 225 MW continuously for 5 hours (for more, refer table 1).
This clear evolution from smaller storage systems to large-scale solar-plus-storage installations highlights how battery advancements have allowed solar energy to become a more reliable and dispatchable resource.
Sources:
- International Energy Agency (IEA). (2020). Energy Technology Perspectives 2020. https://www.iea.org/reports/energy-technology-perspectives-2020
- AFSIA Solar Outlook Report https://www.afsiasolar.com/data-center/outlook-report/
- REPSOL Global https://www.repsol.com/en/energy-and-the-future/future-of-the-world/solar-power-plan
- AVENSTON Classification https://avenston.com/en/articles/pv-power-plants-classification
- “How Wind, Solar, Storage as an Integrated Renewable Hybrid Solution Can Reliably Match Load Requirements”, Amelie Wulff, GE Renewable Energy, Paris, France
- E.I.A., U.S. Energy Information Administration, https://www.eia.gov/todayinenergy/detail.php?id=56880
- Evolution of Li-ion battery price, IEA; Westchester Clean Energy Technologies https://www.westchestercleanenergy.com/post/record-low-lithium-ion-battery-cell-prices
- Evolution of solar PV module cost
- Solar Power Europe, https://www.solarpowereurope.org/press-releases/new-report-global-solar-installations-almost-double-in-2023-but-leaves-emerging-economies-in-the-dark
- TotalEnergies, “South Africa: TotalEnergies Launches Construction of 216 MW Solar Plant,” Available: https://totalenergies.com/media/news/press-releases/south-africa-totalenergies-launches-construction-216-mw-solar-plant.
- Scatec, “Kenhardt 1-3 Solar Hybrid Project,” Available: https://scatec.com/locations/south-africa/#kenhardt-1-3-south-africa-540-mw; Blackridge Research, “Scatec Kenhardt Hybrid Solar Photovoltaic (PV) Farm,” Available: https://www.blackridgeresearch.com/project-profiles/scatec-kenhardt-hybrid-solar-photovoltaic-pv-farm-power-plant-project-northern-cape-south-africa#project-cost.
- Wartsilla, “Case Study: Fekola Hybrid Solar Project,” Available: https://www.wartsila.com/docs/default-source/energy-docs/es-o/project-spotlights/eb-eso-casestudy-af-fekola.pdf?sfvrsn=f7eb1243_6; Baywa re, “Fekola Solar-Plus-Storage at Mali Gold Mine,” Available: https://renewablesnow.com/news/baywa-re-suntrace-fire-up-30-mw-solar-plus-storage-hybrid-at-mali-gold-mine-737657/.
- Eiffage Energy Systems, “RMT Builds a 37.5 MWp Solar Power Plant in Ivory Coast,” Available: https://www.eiffage.com/en/media/news/rmt-builds-a-375-mwp-solar-power-plant-and-installs-an-energy-storage-system-in-ivory-coast-west-africa.
- Energy Storage News, “Globeleq’s Solar-Plus-Storage Plant in Mozambique,” Available: https://www.energy-storage.news/globeleqs-solar-plus-storage-plant-in-mozambique-enters-commercial-operation/; Globeleq, “First Solar-Plus-Storage Plant in Mozambique,” Available: https://globeleq.com/globeleqs-first-combined-solar-battery-storage-plant-officially-begins-commercial-operations-at-cuamba-in-mozambique/.
- Huawei, “Meinergy Signs Agreement with Huawei,” Available: https://solar.huawei.com/en/news-room/en/2022/Meinergy-Signs-Agreement-with-Huawei-on-a-1GW-and-500MWh-Project-of-Ghana; Huawei Case Study, “PV Magazine Special,”.
- Power Africa, “Building Malawi’s First Utility-Scale Solar-Plus-Storage Project,” Available: https://powerafrica.medium.com/building-malawis-first-utility-scale-solar-plus-storage-power-project-6aadd0904727; Afrik21, “Golowmoti Solar Power Plant in Malawi,” Available: https://www.afrik21.africa/en/malawi-golomoti-solar-power-plant-with-storage-goes-into-commercial-operation/.
- ESFA Investment Group, “Noor Ouarzazate: The World’s Largest CSP Plant in Morocco,” Available: https://esfccompany.com/en/articles/solar-energy/noor-ouarzazate-the-world-s-largest-concentrated-solar-power-plant-csp-built-in-morocco/.
- Smart Energy, https://www.smart-energy.com/industry-sectors/storage/iberdrola-commissions-spains-first-solar-pv-plus-storage-plant/
- Energy Storage News, https://www.energy-storage.news/edwards-sanborn-california-solar-storage-project-world-largest-bess-battery-system-fully-online/
- A. Burtin and V. Silva, “Technical and Economic Analysis of the European Electricity System with 60% RES,” EDF Research and Development, June 2015
- A. Adeoye, “Modelling and forecasting hourly electricity demand in West African countries,” in AAM, 2017.
- West African Power Pool (WAPP), “Planning and Prospects for Renewable Energy,” International Renewable Energy Agency (IRENA), 2013.
- National Renewable Energy Laboratory (NREL), “Energy Storage for Grid Resilience and Reliability,” NREL, 2020. [Online]. Available: https://www.nrel.gov/docs/fy20osti/73856.pdf
- Fraunhofer Institute for Solar Energy Systems ISE, “Analysis of Fast Frequency Response Capabilities in Modern Energy Systems,” Fraunhofer ISE, 2020. [Online]. Available: https://publica-rest.fraunhofer.de/server/api/core/bitstreams/a4a6793c-1dfe-4272-ab05-0e6f24604270/content
Other sources used during pre-writing:
- Solar Energy Industries Association (SEIA). (2021). Solar and Energy Storage Technology Overview. https://www.seia.org/initiatives/solar-plus-storage
- BloombergNEF. (2022). Battery Pack Prices Fall to an Average of $132/kWh, But Rising Commodity Prices Start to Bite. Retrieved from https://about.bnef.com/blog/battery-pack-prices-fall-to-an-average-of-132-kwh-but-rising-commodity-prices-start-to-bite/
- U.S. Department of Energy. (2021). Comparative Study on Gas vs. Solar+Battery Systems. Retrieved from https://www.energy.gov/eere/solar/comparative-study-gas-vs-solarbattery
- African Development Bank (AfDB). (2021). Powering Africa’s Future: The Role of Solar Energy. Retrieved from https://www.afdb.org/en/topics-and-sectors/initiatives-partnerships/powering-africas-future
- International Renewable Energy Agency (IRENA). (2023). Renewable Power Generation Costs in 2022. Retrieved from https://www.irena.org/publications/2023/Jul/Renewable-Power-Generation-Costs-in-2022
- World Bank. (2020). The Role of Solar Energy in Bridging Africa’s Electrification Gap. Retrieved from https://www.worldbank.org/en/topic/energy/publication/the-role-of-solar-energy
- Fraunhofer Institute for Solar Energy Systems. (2021). Battery Storage Technologies for a Sustainable Future. Retrieved from https://www.ise.fraunhofer.de/en/research-projects/battery-storage-technologies
- National Renewable Energy Laboratory (NREL). (2022). Advancements in Solar and Battery Storage. Retrieved from https://www.nrel.gov/solar/advancements-in-solar-battery-storage
- BloombergNEF, https://about.bnef.com/blog/lithium-ion-battery-pack-prices-hit-record-low-of-139-kwh/